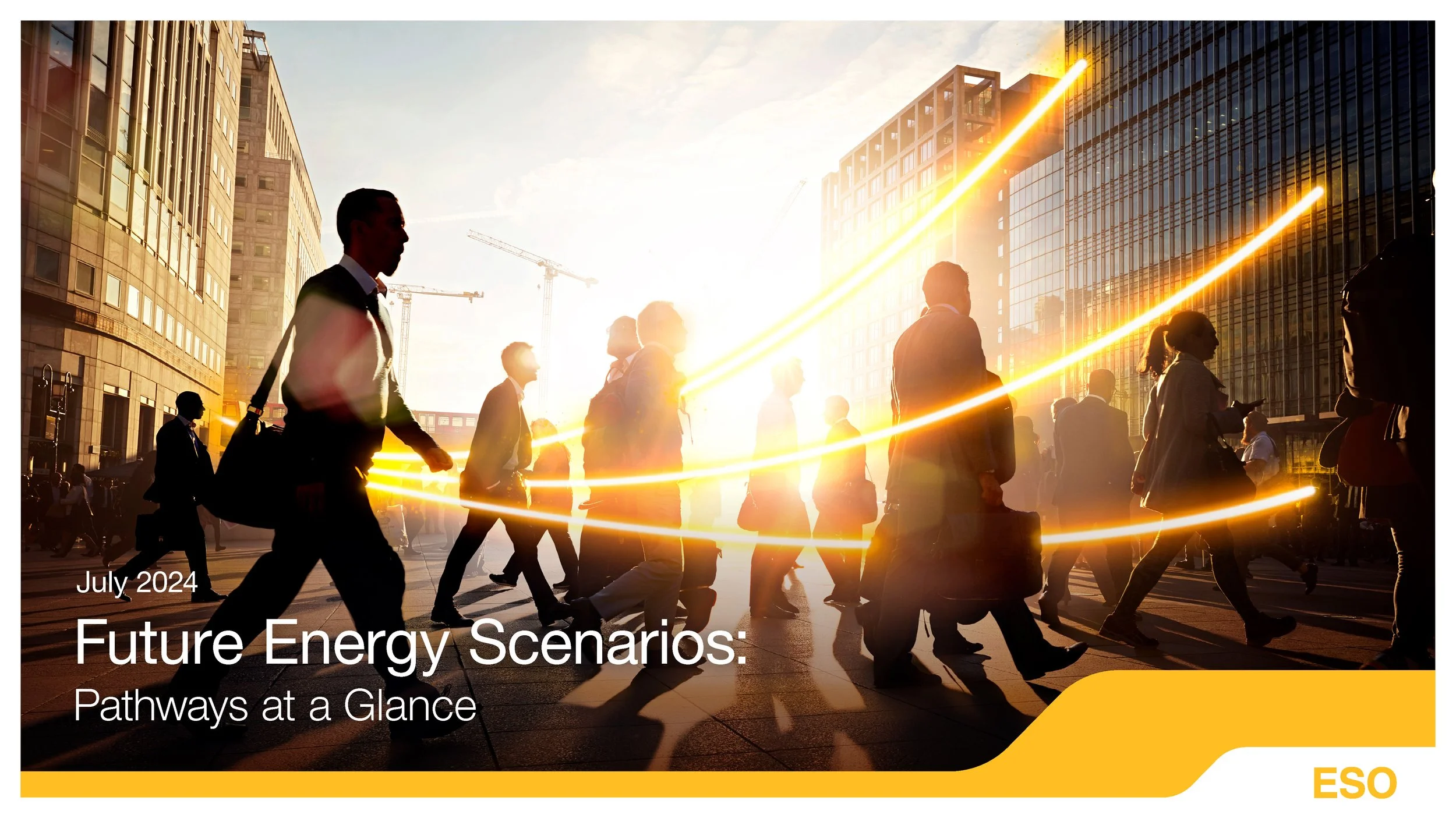
The scale of the challenge.
Energy Supply and demand today and in 2050
Today (above)
Current supplies of energy (type/quantity) are on the left, and their uses on the right. The total annual supply is 1706TWh. UK solar and wind provide 112TWh, or just 6.6% of this total.
2050 (above)
This is just one of three possible future scenarios examined by NG ESO (link below). By 2050 Natural Gas and Other fuels are more or less phased out. Because of energy savings and increasing efficiency the total annual supply is only 1218TWh (a 29% reduction compared with today). UK solar and wind provide 618TWh, or 50.7% of this total.
An Introduction to Renewable Energy
Then…….
We used to produce electricity by burning coil, oil or gas, or from radioactive decay in nuclear power stations. Generating stations occupied small areas of land and the electricity was supplied via a National Grid operating at 400kV - the ‘backbone’ of national supplies - with smaller branches at lower voltages delivering electricity to local substations, ultimately to individual factories or homes. We needed only a few generating stations because the fuel they used had a very high energy content; the grid was relatively simple because of the small number of generating stations and electricity was supplied continuously, 24/7, because the station operators had full control over the supply of fuel to the generators. Just as well, because we need electricity all the time!
Now……
In decarbonising our electricity supply system on the pathway to Net Zero we have closed down most coal-fired stations and are building a range of renewable generating systems, based on solar, wind or tidal energy, retaining gas and nuclear stations to provide the backup or base-load of electricity supplies.
The problem with renewables is three-fold. First, what might be called the ‘energy density’ of renewables is very much less than that of the older coal, oil or gas systems: a very much larger area is required to generate the same amount of electricity. Second, most renewable power is intermittent, not continuous; the sun doesn’t always shine, nor the wind always blow. This has serious implications for our 24/7 demand for electricity and will require very large amounts of energy storage, so that renewable electricity produced in excess of current demand can be stored for later use. Batteries are being added to a number of large-area solar installations, but battery storage is carbon intensive, expensive and limited (generally to no more than about two hours additional output per day). Various methods are suggested for cheap, large capacity, inter-seasonal storage of excess renewable energy, but the UK has not yet embarked on this at the scale that will be required. The third problem of renewables is that they are being installed in places far away from the traditional electricity generating systems and so require additional network connections. Diffuse, intermittent and relatively low output renewable systems will call for a complete revision of our electricity network systems and design. The problem of national intermittent supplies may partly be solved by continental inter-connectors, but this in turn makes us vulnerable to intermittent generators elsewhere, and to terrorists or other ‘bad actors’ cutting the inter-connectors.
In theory - a desktop exercise estimating the UK renewable land requirements.
How would the UK look if all its electricity were produced by renewables? The 2050 Future Energy Scenario at the top of this section suggests that the UK will need 1,218TWh of energy each year, 60% of it (730.8TWh) electricity. How much land or sea area would be required if all of this demand were met by renewable installations? SolarQ has produced the graph on the right, first for the UK as a whole (above). The total land area of the UK (first histogram) is 6.8% urban and the rest non-urban. If all 730.8 TWh of electricity were produced entirely by coal, entirely by nuclear or entirely by biomass burning, their installations would take up about 0.03% of the UK’s total area. For renewables to produce the same total amount of electricity, solar panels would cover just over one twentieth of the country (5.7%), and onshore wind about one sixth (16.8%). The sea area occupied by offshore wind turbines would be equivalent to almost a quarter of the UK land area (21.9%).
The area of England is just over half that of the UK (130,280 sq kms vs 242,500 sq. kms respectively), but it contains just over 85% of the entire UK population. The second graph on the right (lower) shows the results assuming England generated 85% of the total UK electricity requirements on England’s smaller land area. All percentages are obviously higher, with the area for ground-based solar rising to 9.1% of the total, the on- and offshore wind figures rising to 26.7% and 34.7% respectively.
Conclusions, this section
This desktop exercise shows that biomass burning has about the same performance in terms of energy density as the traditional, polluting coal generators. The biomass figures here were based on information from the Drax power station in North Yorkshire, UK, the largest biomass installation in Europe. Drax burns 20,000 tonnes of wood pellets each day, all imported from North America (USA and Canada) or Europe, and generates 14TWh of electricity per year. It is the single largest emitter of CO2 in the country, and generates about 4% of the UK’s electricity supply (all sources). Controversially it is also officially considered a renewable energy resource (the CO2 released will eventually be absorbed by new planted trees but this will take decades) and is said to contribute up to 17% of the country’s renewable electricity output. In the absence of significant Carbon Capture from Drax’s biomass burning, however, the generator should not be considered as a renewable energy source. Almost total reliance on overseas supplies of wood also means the nation will never be self sufficient in biomass-produced electricity.
For the UK as a whole, and also for England alone, solar panels would need to cover an area about equivalent to all our current urban cover (this includes houses, roads etc.). Ground-mounted solar panels as currently being installed in the UK (at relatively low height above ground level) preclude agriculture from being carried out under them. Agri-voltaics, practised elsewhere, raise the panels several metres off the ground so that farm machinery can harvest crops beneath; but this requires much stronger and more robust staging to stop the panels toppling over in high winds. The introduction of sheep, ducks etc beneath solar panels in the UK is being called ‘agri-voltaics’, but hardly deserves this description. These animals are being used to keep the vegetation down around and beneath the panels (which would otherwise involve mowing or herbicides) and are therefore a necessity rather than a virtue.
The calculations suggest that a very large area would be required for both on- and offshore turbines to meet the UK’s total electricity requirements. The figures represent the areas within the redline boundaries of the wind installations - the plan sites. The wind industry claims that only about 1% of the totals here are the areas required for the support towers - the masts (the total land cover just for the masts is indicated by the ‘Turbines’ call-out in the histograms above: 0.15% for the UK and 0.24% for England). The turbine blades are set well above ground level so that ‘normal agriculture’ can be practised between on-shore wind turbines. Within any one wind farm, the turbines are spaced far apart so that the turbulence of one turbine does not affect the efficiency of its neighbours.
Large spacing between turbines isn’t a problem for offshore wind installations. By not taking up valuable land they are perhaps an obvious choice for an over-crowded island; their high Load Factors (see below) make them particularly attractive.
Intermittency, Load Factors and Net Carbon performance
A good, environmentally friendly renewable energy source would be one generating as much electricity as possible, for as much of the day/night as possible, and at the lowest ‘carbon cost’ possible. This section looks at the performance of the renewable energy sources we have at present.
A renewable energy source has an installed ‘nameplate’ capacity that represents the greatest possible output from that source under ideal conditions. Thus, for example, a 400Watt solar panel can produce no more than 400Watts of output on the brightest day. That figure is determined under laboratory conditions where light of 1000Watts per sq. metre (the maximum possible level of the sun’s radiation at the earth’s surface) is shone on the solar panel and the output power from the panel (amps, volts) is determined. One measure of the Efficiency of solar panels is the ratio of Watts out/Watts in, measured in those laboratory conditions. For the current generation of solar panels, efficiency is about 20% but new technologies are improving this all the time. That measure of efficiency is ‘baked into’ the nameplate capacity printed on the side of the solar panel and will not concern us further here. Needless to say, if two panels of the same physical size have different nameplate capacities, the one with the larger capacity has a higher efficiency.
But a 400Watt nameplate capacity solar panel rarely produces 400Watts of output power because the sun doesn’t shine brightly enough all the time; in fact it shines only rarely for that maximum output to be achieved. This is the problem of Intermittency that affects all types of renewable energy. The proportion or percentage of the total time that any electricity generator produces its nameplate (i.e. maximum) output is called the Load Factor and Load Factors are very important in the renewable energy debate.
The graph on the right shows Load Factors for a variety of renewable electricity sources in the UK, by quarterly periods from 2017 to 2020. The average figures given there (in the bubble call-outs) are those for the 10 year period (measured on a month-by-month basis) from 2012 to 2021. These are official figures from the UK’s DBEIS Digest of UK Energy Statistics (DUKES). Solar panels have load factors of about 11%. On- and off-shore wind turbines have Load Factors of 26.6% and 39.2% respectively. Put simply, on our small temperate island the wind blows more than the sun shines. That probably comes as no surprise to anyone. But importantly here the wind blows more in Winter than in Summer and hence wind turbines produce more electricity in Winter (Load Factors above 50% - see graph), just when we need it. Solar panels produce more in Summer than Winter. In fact Winter performance of solar panels in the UK is abysmal (explored in more detail in one of our Reports in the Analysis section). While there are no economies of scale in the load factor of solar panels (larger installations have the same load factor) offshore wind-turbines are gradually increasing in size and a 2023 report imagines off-shore wind Load Factors of 69% by 2036.
Wind is Best?
Don’t take our word for it. Listen to the expert. Australian Professor Martin Green, 2023 Winner of the Queen Elizabeth Prize for Engineering for increasing the efficiency of Solar Panels, said on the day he received his award:
”But I think wind is the real solution for the UK, whereas in Australia solar is going ahead leaps and bounds".
Interpretation of Load Factors
Load Factors have caused a great deal of un-necessary confusion. A Load Factor of 10% means that the device is working at its maximum possible output for the equivalent of 10% of the time, or 2.4 hours in each 24hr period. It does not mean it is working for only 2.4 hours a day and not at all for the other 21.6 hours a day. For example, it could be working at half its maximum capacity for 4.8 hours a day. From the point of view of the power output from the system - the total Watt hours generated per day - the result is exactly the same. And it is Watt hours that electricity supply companies are interested in because demand is also in Watt hours. When the Watts generated in any one hour of the day do not coincide with the demand in Watts from consumers for that same hour of the day, alternative sources of electricity must be provided. The mismatch of supply of electricity from renewables and the demand of electricity by consumers becomes more problematic the more intermittent the renewable supply. Put another way, a system relying on a renewable source which has a low load factor will require more energy storage than one with a higher load factor. This explains why we have not needed much energy storage for our traditional supply systems (coal, oil, gas) which have high load factors (and where down-time for maintenance can be scheduled and alternative supplies brought on-stream).
Where is the UK in the list of countries for their Solar potential?
In 2020 the World Bank produced a report that ranked the world’s 240 countries for their solar photovoltaic (PV) potential. The results are shown in the clip below. The scale of 1 to 7 at the top and bottom of the clip are the hours per day for maximum performance of solar PV panels in each country (i.e. they are the Load Factor expressed as hours per day). Click on the clip to start it.
The countries scroll up the screen in rank order, the best (Namibia) first. The scrolling list has all the country names on the left - too small to read here - and then the mean ‘hours per day’ of bright sunshine on the right; the brown bars to the left and right of the mean values indicate variability around the mean. Namibia experiences between 5 and 6 hours of bright sunshine each day (i.e. a solar PV Load Factor of 23%). As the list scrolls up the screen, key countries are indicated by call-outs on the right. Professor Green’s Australia is number 54 in the list (not yet indicated by a call-out). Understandably, high latitude countries appear lower down the list.
But where is the UK? You have to wait until the very end to see that the UK is ranked 239 out of the world’s 240 countries for its solar PV potential (2.64 hours of bright sunshine each day; Load factor 11%), beaten from last place only by even cloudier Ireland. If it’s sun you want, don’t go to Ireland for your holidays!
Conclusion, this section
All other things being equal, it appears that for the UK wind turbines are a better source of renewable energy than are solar panels. They have higher load factors, produce more electricity when it’s needed (in Winter), and - because of those load factors - are less intermittent, requiring less long-term storage. Their obvious draw-back is the large areas they need, something not so important for offshore wind but which may be important on-shore. Although wind turbine masts occupy only a small fraction (1%) of the total area of the installation (allowing regular agriculture to be carried out between the turbines), the visual impact of large rotating structures can be unwelcome to local communities. Negative impacts of wind farms on house values have been reported and stretch over longer distances than similar impacts of solar farms.
The great Carbon debate. Which renewable energy source is cleaner and greener than all the others?
Renewable energy projects usually claim that the new technology proposed (solar, wind etc.) will avoid the emission of so many tonnes of carbon that would otherwise be emitted from traditional sources generating the same amount of electricity. Detailed calculations are rarely shown and may be ‘massaged’ assuming traditional supply from burning dirty coal rather than cleaner gas, thus putting the proposal in an even more favourable light.
Carbon pay-back times assume that the mining, manufacture, construction and installation of a renewable energy source all involve the release of CO2 into the atmosphere and then ask how long does the (non-CO2 producing) renewable resource have to run before the clean energy it produces has avoided the release of that same amount of CO2 by traditional electricity generators. Once again, figures can be made to look more favourable by imagining a particularly polluting traditional electricity generator.
But neither of the above methods measures the relative carbon efficiency of different renewable electricity generating methods. Surely we should be using renewable systems that generate the greatest amount of electricity at the lowest possible carbon cost to the environment? To compare systems in this way we need two pieces of information; first the total CO2 released during mining, manufacture, installation, running & maintenance and final decommissioning of any renewable system (its ‘Total Life Cycle’ carbon dioxide, or TLC) and second the total amount of electricity (kWh) generated by that system during its operational lifetime. Dividing the first number by the second tells us the amount of CO2 emitted per kWh of electricity generated throughout the system’s lifetime. The lower the resulting figure, the better, or ‘greener’ the generating system.
The American National Renewable Energy Laboratory (NREL) has done these calculations for a variety of renewables with the results shown in the Table on the right (click here for the original). The ‘One-time Upstream’ costs are the amounts of CO2 released in mining, manufacturing and installing each system. The ‘Ongoing Non-Combustion costs’ are those for operation and maintenance of the system as it produces electricity. Finally the ‘One-time Downstream’ costs are those for decommissioning each system (figures in each case are gCO2 released per kWh). The Total Life Cycle costs are the sum of those three figures. The TLC costs for solar (43gCO2e/kWh) are more than three times those for wind (13gCO2e/kWh), the figures for which are for a combination of on- and off-shore installations; the offshore figure would be even more favourable. In fact, solar is the most polluting of all the renewables in that Table, bar biomass burning (52gCO2/kWh) which we do not consider renewable anyway.
The above calculations become irrelevant when all of our mining, manufacturing etc. processes are powered by renewable, non-polluting systems. There will then be no carbon costs of production etc. But we are not there yet. If we want to end up with the greenest future, shouldn’t we choose the greenest pathway to get there?
Total Life Cycle Carbon - the Video!
The short video on the right helps to explain the difference in the TLC figures for Solar and Wind renewable systems (click on the clip to start). First the TLC equation is developed at the top. Then each system starts to generate electricity, as shown by the meters above each picture. Each unit of electricity from solar involves the release of 3 times more CO2 than each unit from wind. Later in the clip, night falls and solar ceases to produce anything. But the wind turbines keep turning, generating electricity.
Once again the key here is the Load Factor. No matter how much or little CO2 is involved in the construction of a renewable system, if it doesn’t produce any electricity for much of its operational lifetime (i.e. if it has a low Load Factor) its TLC/kWh figure will be high.
Conclusion, this section
Although the Total Life Cycle figures for biomass generators are perhaps not surprising those for solar are. In temperate regions the sun doesn’t shine enough to improve the TLC figures any further. Solar’s advantage is that it can be easily installed at relatively modest capital cost with low levels of technological expertise. Its great advantage is that the returns on capital - a function of the price UK consumers pay for their electricity - are currently large. The present generation of ground-mounted solar applications mention lifetimes of 30 or more years (up to 60 years have been quoted), frequently still claimed to be only ‘temporary’, but no commercial solar panel available to date has a guarantee of more than 30 years. Through clauses in the NPPF that mention ‘life extension’ or ‘re-purposing’ of renewable installations the stage is set for more or less permanent loss of farmland to solar. But within 30 years surely there will be new, zero carbon technological revolutions (including nuclear fusion) rendering large-area renewable energy generation obsolete?